Explore the interactive timeline to discover key milestones and achievements in the journey of CERN. Navigate through the years to see our history come to life, click on each title to find out more.
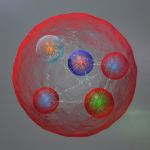
First observation of pentaquarks at the LHC
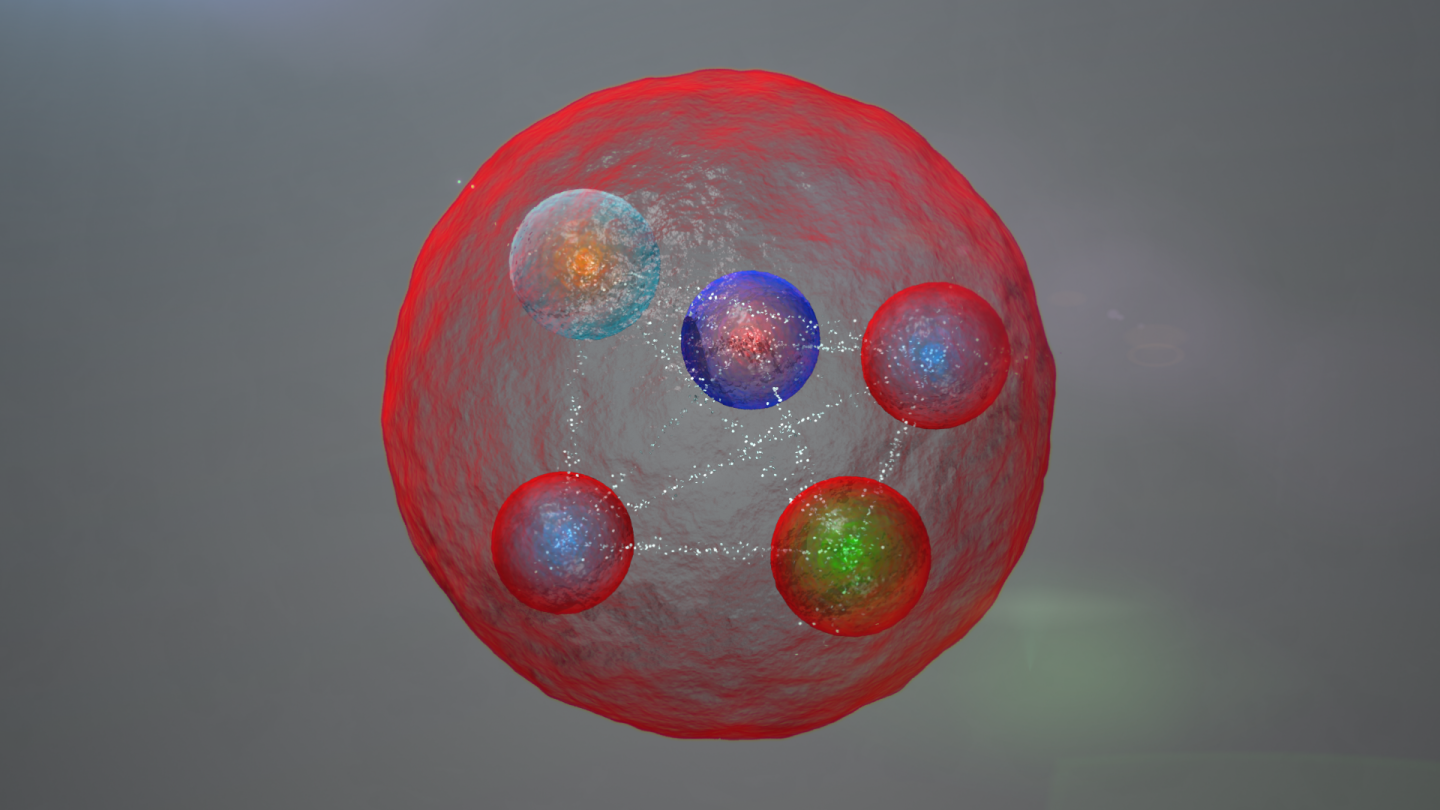
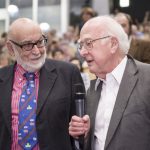
Nobel Prize in Physics for the prediction of the Higgs field
One year after the discovery of the Higgs boson, François Englert and Peter Higgs are awarded the 2013 Nobel Prize in Physics for the theoretical prediction they made decades earlier, together with the late Robert Brout, of a new pervasive fundamental field in the Universe. This field, known as the Higgs field, manifests itself through the Higgs boson and gives mass to elementary particles through the Brout-Englert-Higgs mechanism.
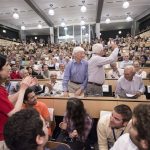
ATLAS and CMS discover a particle consistent with the Higgs boson
On 4 July, the ATLAS and CMS collaborations announce the discovery of a particle with features consistent with those of the Higgs boson, a particle predicted almost 50 years earlier. This particle is a manifestation of the Higgs field, which gives mass to elementary particles. The following March, after examining two and a half times more data, ATLAS and CMS conclude that some kind of Higgs boson has indeed been discovered.
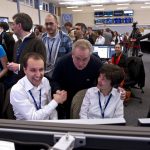
The LHC delivers its first physics collisions
The Large Hadron Collider (LHC) physics programme starts with protonâproton collisions at the unprecedented collision energy of 7 TeV (3.5 TeV per beam), recorded by the four largest LHC experiments â ALICE, ATLAS, CMS and LHCb. Later in the year, lead ions are delivered to the experiments for the first-ever heavy-ion run at the LHC. Since then, the energy of the LHC collisions has constantly increased to achieve a record-energy of 13.6 TeV in 2022.
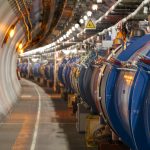
The Large Hadron Collider starts up
On 10 September, at 10.28 CEST, a beam of protons is successfully steered around the 27-kilometre Large Hadron Collider for the first time. This is the culmination of an extraordinary technological and industrial effort to build the world’s largest superconducting machine, cooled to -271°C, only two degrees above absolute zero. A few days after the start-up, an incident damages 53 magnets, halting the machine for several months. The LHC starts up again in 2009, producing its first collisions in December of that year.
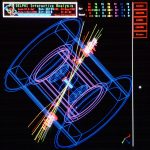
The legacy of CERNâs first big precision machine
The Large Electron-Positron (LEP) collider is decommissioned in November. Over its 11 years of operation, from 1989 to 2000, it has produced 17 million Z bosons, uncharged mediators of the weak force, and approximately 40Â 000 pairs of W bosons, charged mediators of the weak force. The precision measurements conducted at LEP have provided stringent tests of the Standard Model of particle physics, confirming the existence of three generations of fundamental particles and placing the Standard Model on solid experimental ground.
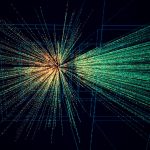
First evidence of QuarkâGluon Plasma
A combination of results from several experiments provides evidence for the existence of Quarkâgluon plasma (QGP), a state of matter believed to have existed just after the Big Bang. The experiments all measured different aspects of collisions between the nuclei of heavy atoms and a fixed target at the Super Proton Synchrotron (SPS). This result is the culmination of a heavyâion programme that started in 1986.
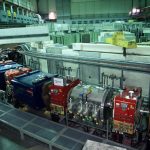
Antiproton Decelerator starts up
The Antiproton Decelerator (AD) starts delivering low-energy antiprotons to antimatter experiments. This 188-metre-long machine decelerates antiprotons so that they can be trapped by experiments. The aim is to study antimatter in depth to determine any difference compared to ordinary matter. In 2017, a newer deceleration ring, ELENA (Extra Low ENergy Antiproton), is connected to the AD to further reduce the speed of the antiprotons.
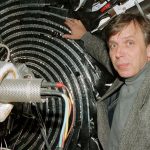
First-ever anti-atoms produced at CERN
An experiment led by Walter Oelert creates nine atoms of antihydrogen. This is the first time that antimatter particles are brought together to make complete atoms.
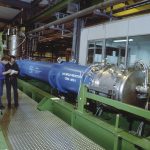
The Large Hadron Collider gets the green light
The Large Hadron Collider project is approved in December. The successful tests of several superconducting magnets demonstrate the feasibility of this innovative machine. The civil engineering works to build the new service tunnels and experimental caverns start in 1998 and are completed in 2005. The development and production of the LHC components, including the impressive magnet system made of thousands of huge superconducting magnets, starts all over the world.
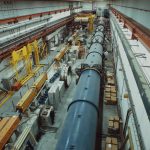
First results on direct charge-parity symmetry breaking are published
The NA31 experiment, using a proton beam from the Super Proton Synchrotron (SPS), publishes the first precise results on what is known as âdirectâ charge-parity (CP) symmetry breaking. This discovery contributes to explaining the tiny difference in the behaviour of matter and antimatter particles. The measurement is later further refined by the NA48 experiment, running at the SPS between 1997 and 2001, as well as by the KTeV experiment at Fermilab in the United States.
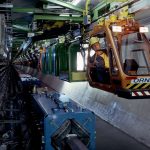
The largest instrument ever built for research begins operation
The Large Electron-Positron collider (LEP) is commissioned in July 1989. With its 27-km circumference, the LEP is the largest accelerator ever built. Fed by CERNâs accelerator complex, it provides particle collisions to four enormous detectors â ALEPH, DELPHI, L3, and OPAL.
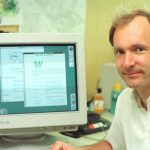
Tim Berners-Lee invents the World Wide Web
Tim Berners-Lee, a computer scientist at CERN, submits the first proposal for the World Wide Web in March. His idea is to merge data networks and hypertext together in a powerful and easy-to-use global information system. By the end of 1990, the first Web server and browser is up and running. In 1993, CERN makes the source code of the World Wide Web available on a royalty-free basis. By the end of 1994, the Web already has 10 000 servers and 10 million users.
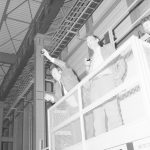
Nobel Prize in Physics for the discovery of the W and Z bosons
Only a year after the discovery of the W and Z bosons, the two key scientists behind it receive the Nobel Prize in Physics. Carlo Rubbia, the instigator of the SPS acceleratorâs conversion into a proton-antiproton collider and spokesperson of the UA1 experiment, shares the prize with Simon van der Meer, inventor of the stochastic cooling technique, which is vital to the collider’s operation.
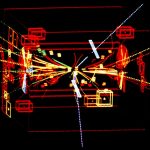
Discovery of the W and Z particles
On 25 January, CERNâs UA1 and UA2 experiments announce the discovery of the W boson, the particle that mediates the weak force, marking a significant milestone in particle physics. This discovery at the Super Proton Synchrotron (SPS) is followed a few months later by that of the Z boson, completing the experimental verification of the electroweak interaction theory.
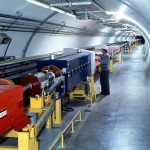
CERNâs 7-kilometre accelerator is switched on
The first beam of protons circulates in the full 7 km of the Super Proton Synchrotron (SPS) on 3 May. This accelerator has since gone on to become the workhorse of CERNâs particle physics programme. Just a few years later, the SPS is converted into a proton-antiproton collider, leading to the Nobel-Prize-winning discovery of the W and Z particles. In 1989, it begins serving also as an injector for a larger machine, the Large Electron-Positron Collider (LEP).
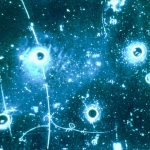
The discovery of neutral currents
The Gargamelle experiment discovers neutral currents, a phenomenon that provides key evidence supporting the electroweak theory. This theory unifies the weak force with the electromagnetic force in a single interaction. This major discovery in the understanding of particles and forces paves the way for the hunt for the W and Z bosons, the particle mediators of the weak force.
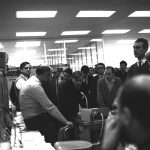
First-ever protonâproton collisions
The worldâs first collisions between two beams of protons occur in the Intersecting Storage Rings (ISR). During its 13 years of service, the ISR makes important contributions to the understanding of the constituents of the proton and probes the strong nuclear force that links binds quarks and gluons together inside protons and neutrons. It also becomes the first proton-antiproton collider and the first ion collider, enabling CERN to gain unique expertise for its subsequent particle collider projects.
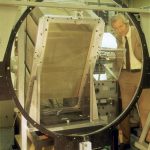
Georges Charpak revolutionises particle detection
CERN physicist Georges Charpak invents the multiwire proportional chamber, a device which revolutionises the recording of particle collisions by capturing them electronically instead of optically. This innovation transforms particle physics, dramatically increasing the number of collisions that can be analysed and allowing rare phenomena to be studied. Charpak receives the 1992 Nobel Prize in Physics for this invention.
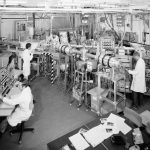
The nuclear research facility at CERN receives its first beams
The Isotope Separator On-Line Device (ISOLDE), a nuclear research experiment, comes into service in October. Over the years, ISOLDE has grown to provide beams of atomic nuclei for several dozen experiments annually. Fundamental research at ISOLDE ranges from studies of the structure of atomic nuclei to materials science, radiation protection, life sciences and astrophysics.
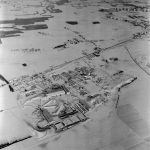
The Laboratory crosses the border
On 13 September, the French and Swiss states sign an agreement to extend the CERN site across the French-Swiss border, in preparation for the construction of a new machine â the Intersecting Storage Rings (ISR) â due to start the following year. With this expansion, CERN becomes the only research centre in the world to straddle the border of two countries.
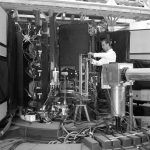
First observations of antinuclei
A team led by Antonino Zichichi using the Proton Synchrotron at CERN, and a team led by Leon Lederman at the Brookhaven National Laboratory (USA), simultaneously observe the antideuteron, the antimatter nucleus composed of an antiproton and an antineutron. This discovery is an important step in understanding how antimatter behaves.
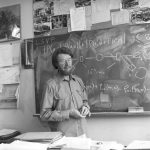
Probing quantum physics
CERN theorist John Bell formulates a theorem that makes an essential contribution to quantum mechanics. The mathematical criteria derived from this theorem, known as Bell’s inequalities, enable quantum entanglement to be tested. Over the following decades, Bellâs work has a profound impact on the development of quantum technologies, such as quantum computing and cryptography.
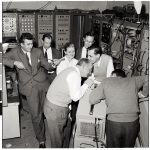
CERNâs first big particle accelerator starts up
The Proton Synchrotron (PS) accelerates protons for the first time on 24 November, at the energy of 24 GeV, briefly becoming the worldâs highestâenergy particle accelerator. With a circumference of 628 metres, it is CERNâs first big particle accelerator. The PS continues to provide beams for experiments to this day, in addition to being an injector for larger machines.
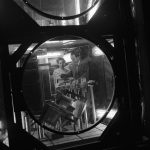
CERNâs first discovery
Using the proton beam from the Synchrocyclotron (SC) accelerator, CERNâs first experiment discovers a rare process: the decay of a particle known as the pion into an electron and a neutrino, in accordance with the predictions of the weak interaction theory. This first discovery is made only one month after the start-up of the experiment.
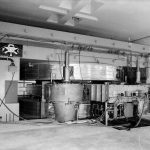
CERNâs first accelerator begins operations
In 1957, the Synchrocyclotron (SC), a 600 MeV proton accelerator, is CERNâs first accelerator to begin operation, providing beams for the Laboratoryâs first experiments from 1958 onwards. In 1967, it begins supplying particles for a dedicated nuclear research facility called ISOLDE. The SC is decommissioned in 1990.
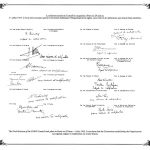
The European Council for Nuclear Research is born
The Convention establishing the Organization was signed at the sixth session of CERN’s provisional Council, held in Paris in the summer of 1953. It was gradually ratified by the twelve founding Member States: Belgium, Denmark, France, the Federal Republic of Germany, Greece, Italy, the Netherlands, Norway, Sweden, Switzerland, the United Kingdom and Yugoslavia. On 29 September 1954, the European Council for Nuclear Research officially comes into being. The provisional CERN Council is dissolved, but the acronym remains.
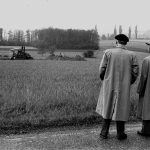
Breaking ground
On 17 May, the first shovel of earth is dug on the Meyrin site in Switzerland, in the presence of Geneva officials and members of CERN staff. Geneva had been selected as the site for the Laboratory, from among four other cities, at the third session of the provisional Council in 1952, following a referendum in the canton of Geneva in 1953.
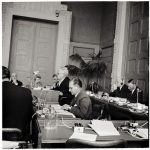
Early beginnings
The first meeting of the CERN Council takes place at UNESCO in May 1952, with Swiss physicist Paul Scherrer in the chair. Among the other roles assigned at the meeting, Edoardo Amaldi is made Secretary-General of the provisional organisation.
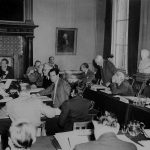
Origins
French physicist Louis de Broglie puts forward the first official proposal for the creation of a European laboratory during the European Cultural Conference held in Lausanne (Switzerland) in December 1949. At the UNESCO Intergovernmental Conference held in Paris in December 1951, the first resolution to create a European Council for Nuclear Research (CERN) is adopted. Two months later, eleven countries sign an agreement establishing the provisional CERN Council.