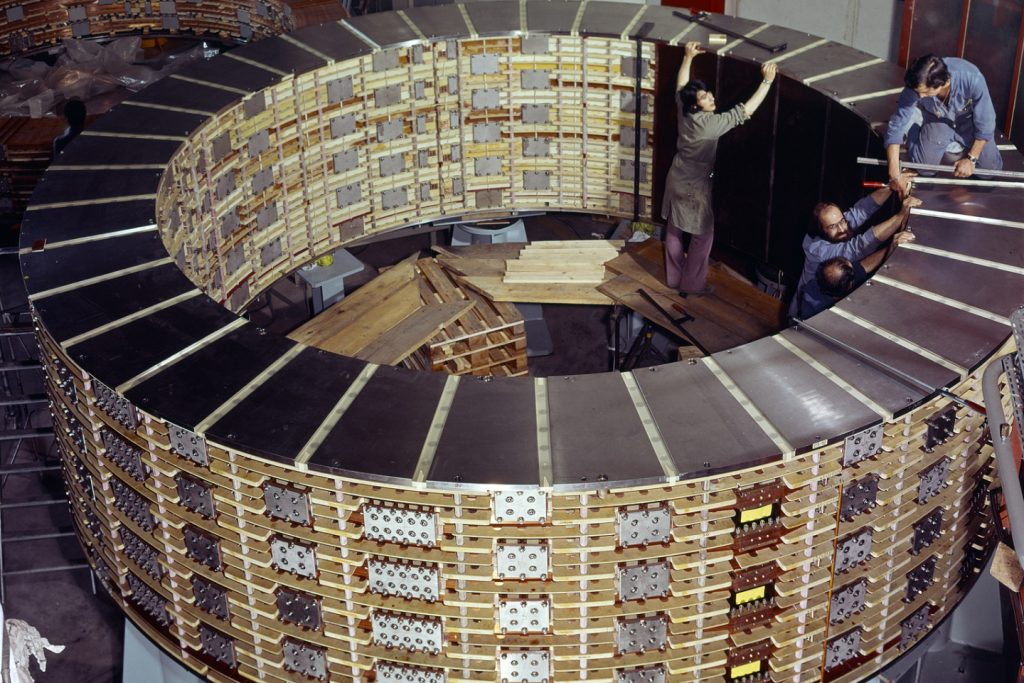
The LHC, the largest superconducting machine in the world, demonstrates how particle physics and CERN have been a driving force in the development of superconductors.
Superconductivity quickly emerged as a very useful property for high-energy physics. Since superconductors lose all electrical resistance below a certain, very low temperature, they are able to transport very high electrical currents, which makes it possible to produce magnets that generate strong magnetic fields, as well as powerful accelerating cavities.
Detectors were equipped with the first superconducting magnets from the 1960s onwards. In 1965, an experiment at CERNās Synchrocyclotron began testing a small superconducting coil that generated a magnetic field of 4.2 teslas. A few years later, in 1973, the Big European Bubble Chamber (BEBC) was fitted with a huge superconducting magnet of over three metres in diameter, a record at the time.
Detectors have progressively been equipped with increasingly powerful magnets, up to the scale of the giant ones in use at the LHC. CMS boasts the largest superconducting solenoid in the world and ATLAS the largest toroidal magnet, made up of 25-metre-long coils.
The magnets that are destined for use in accelerators must be produced in their dozens, their hundreds or even their thousands, which explains why it took until the 1980s for the first superconducting accelerators to come into service. The Tevatron collider in the USA, which came online in 1983, was the first large-scale application of these magnets. In 1989, the Large Electron-Positron Collider (LEP) at CERN was equipped with a few superconducting magnets designed to focus the bunches of particles before they collided. But, above all, superconducting accelerating cavities were installed in the LEP from 1996 onwards: more than 280 in number, they were capable of generating more intense accelerating fields to increase the energy of the collider.
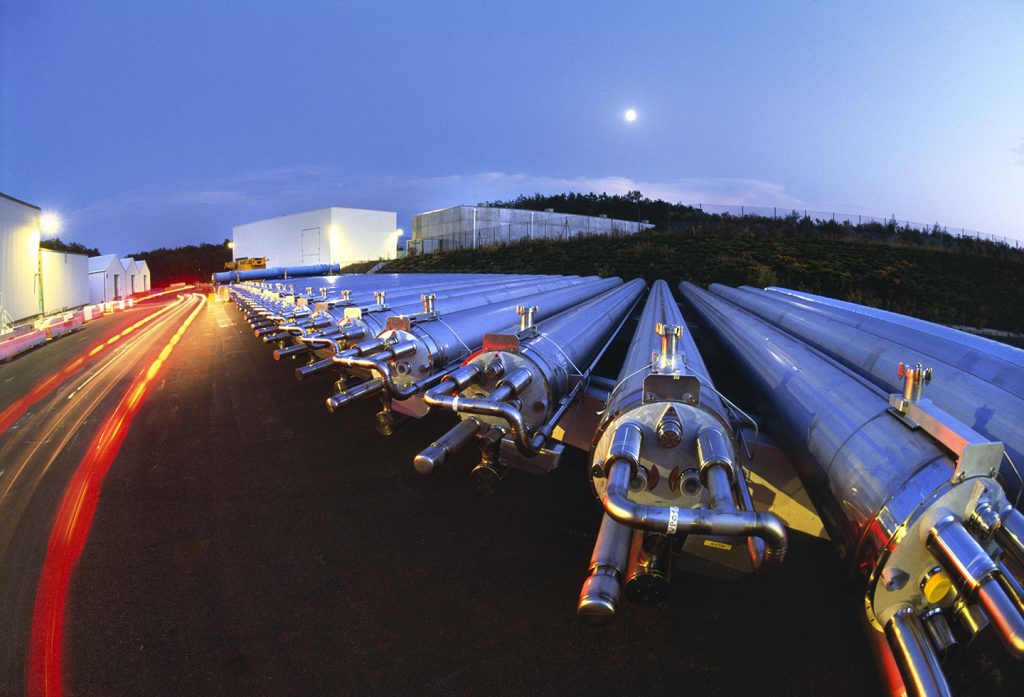
Even before the LEP came into service, the teams at CERN were already working on its successor, a proton collider to be installed in the same tunnel, based on superconducting magnets generating fields of more than 8 teslas. The epic technological and industrial task of creating the largest superconducting facility in the world would take 25 years to complete. This collider, the LHC, contains more than 9000 niobium-titanium superconducting magnets, including 1232 15-metre-long dipole magnets that each weigh 35 tonnes. Of the acceleratorās 27 km circumference, 23 km are cooled with superfluid helium to 1.9 degrees above absolute zero (-271 °C).
The LHC has also prompted work on high-temperature superconductors, which do not have to be cooled to such glacial temperatures. The LHC current leads ā the connections in which the current passes from the ambient temperature to the temperature of the liquid helium in the magnets ā are made of these superconductors, which are ceramic and very complicated to deploy.
The High-Luminosity LHC, the major upgrade of the LHC that is currently being built, also brings its own share of innovations in the field of superconductors. It will be equipped with magnets made from a superconducting niobium-tin compound, which will produce more intense magnetic fields of 12 teslas. It will also use electrical connections made from intermediate-temperature superconductors, which can operate at 25 kelvins (-248°C). The CERN teams are continuing their work on high-temperature superconductors, the Holy Grail for experts in this field, which, in the longer term, could open up unprecedented possibilities for fundamental physics and have numerous applications for the benefit of society in general.
Recollections
In 2000, I [was asked] if I would consider leading CERNās team in charge of superconducting magnets. It took me approximately one microsecond to say yes! Then started this incredible adventure of building the most complex accelerator in the world, using the most complex technology.
Lucio Rossi
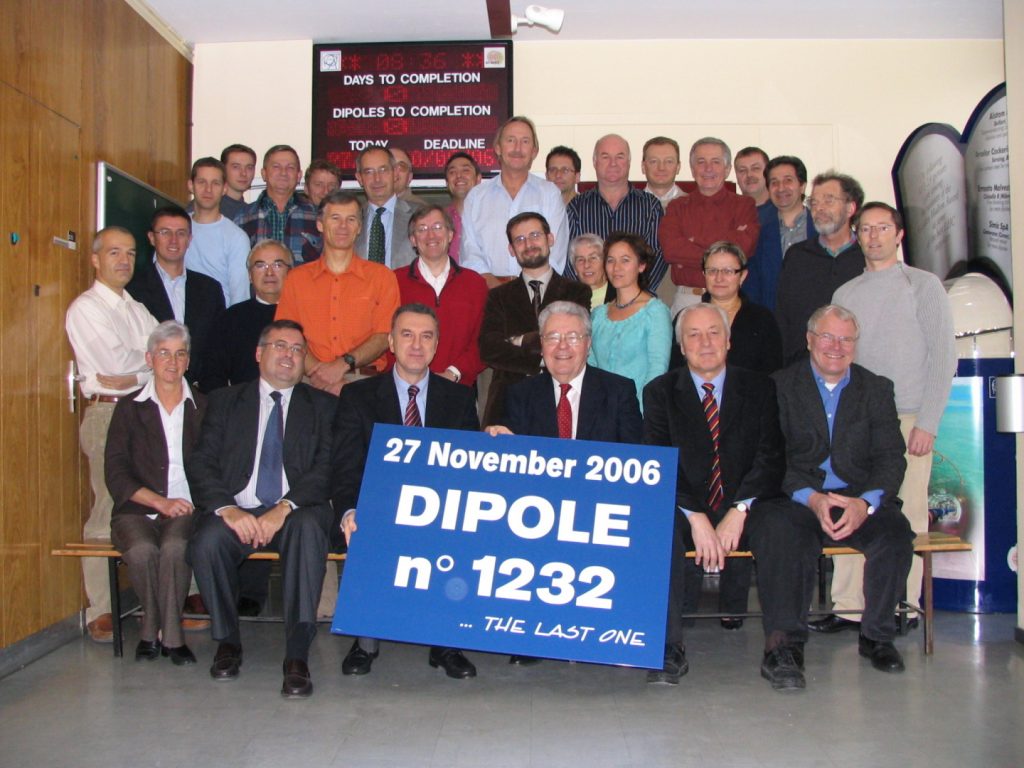
Lucio Rossi began his career in plasma physics and moved into the field of applied superconductivity for accelerators in the early 1990s, heading the INFN-LASA team that had just begun to work on the first prototype superconducting dipole magnet for the LHC. In 2001, he became the leader of CERNās Superconducting Magnets and Cryostats group, which was responsible for developing and launching the industrial production of the superconducting magnets needed for the LHC. From 2010 to 2020, he was the leader of the High-Luminosity LHC project.
āThe INFN-LASA team delivered the first LHC prototype magnet to CERN in 1994. This type of magnet has been very successful and that first dipole was used until recently by the CAST experiment ā you could still read the words āLHC DIPOLE PROTOTYPE CERN-INFN 1ā on it.
A few years later, in 2000, I received a phone call from Lyn Evans, then leader of the LHC project, who asked me if I would consider leading CERNās team in charge of superconducting magnets. It took me approximately one microsecond to say yes! Then started this incredible adventure of building the most complex accelerator in the world, using the most complex technology. We had to produce 300 000 km of superconducting wire for the LHC superconducting dipole and quadrupole magnets and for the several thousand corrector magnets, and to do so we had to collaborate with industry. That was not easy. Moving from our complex superconducting dipole magnet prototypes to industrialisation was a challenge, in particular when it came to getting the level of quality that we wanted from the companies. We had to make compromises, because sometimes best is the enemy of good. It was difficult, but we did it.
Producing the magnets was a real challenge; assembling them and making them work together was yet another one. The difficulty with the LHC is that everything is in series, so if you have a single weak link, the chain breaks. And thatās what happened on 19 September 2008. One single electrical connection between two adjacent magnets ā out of 10 000 such connections ā failed, leading to extensive collateral damage and a one-year delay. I have to assume that the mistake was made by us, the engineers, the designers, because we had thought of a system that was not robust against faulty workmanship.
We learnt from this incident collectively. I think this is the strength of CERN: at that moment, I could feel that CERN was united. Afterwards, the LHC was resurrected stronger than before, and we got the Higgs boson.
When the LHC restarted, in November 2009, it was really a triumphal march. I was very moved to see the LHC restarting, to see the machine working well. We had done a good job. That was a glorious day.
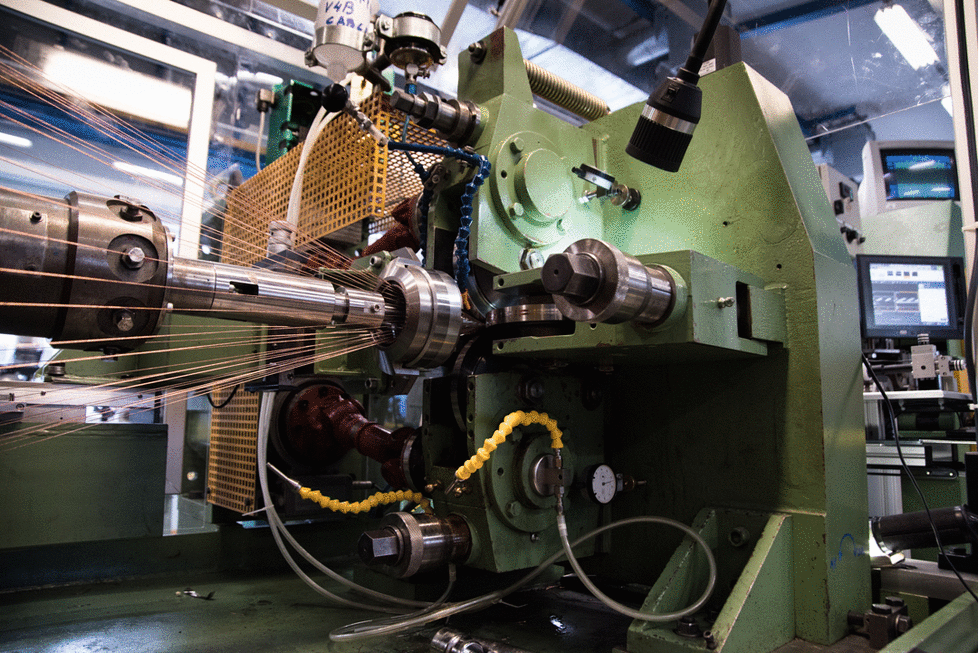
In 2010, we then started to think about the future of CERN, and I proposed the High-Luminosity LHC project. This was an important project for me, as the HL-LHC sort of closed the loop for me personally, so I took on the role of HL-LHC Project Coordinator in 2010. From then on, we flew… but not without some difficulties, as one can expect in such a project, the biggest one probably being COVID-19. We also had some difficulties with the magnets: for the HL-LHC, we decided to build new types of magnets, based on the new niobium-tin superconducting technology, while the LHCās magnets are based on the niobium-titanium technology.
We thought that we would set up the technology on a short magnet, a 1.5-metre-long magnet, and then just extend it to a 7-metre-long magnet. It was like that for the LHC. But we learnt the hard way that it was not so easy with the niobium-tin technology, as the coils are much more fragile; thatās why we faced these challenges, in particular with the HL-LHC 11 T magnets. We also learnt from failures, and we now have successful long magnets for the inner triplet quadrupoles, a key element of the HL-LHC, but it took a lot of time. That was a bumpy ride but it was necessary. The HL-LHC will be a real test of the niobium-tin technology, to help make the jump to future colliders.
CERN is here to make things that are truly difficult, to dream what is not yet possible. I’m convinced of this, otherwise it wouldnāt be CERN.ā